INTRODUCTION
MATERIALS AND METHODS
Plant materials and extracts
Salt stress treatments
Rice growth and relative water contents after salt stress
Photosynthetic efficiency, chlorophyll and carotenoid contents after salt stress
Determination of superoxide radical (O2−) and H2O2 contents after salt stress
Lipid peroxidation after salt stress
Proline and sugar accumulation after salt stress
Statistical analysis
RESULTAS AND DISCUSSION
Effects of plant extracts on rice injury and growth under salt stress
Effects of selected plant extracts on relative water content under salt stress
Effects of selected plant extracts on photosynthetic efficiency and pigments under salt stress
Effects of selected plant extracts on reactive oxygen species and lipid peroxidation under salt stress
Effects of selected plant extracts on proline and sugar accumulation under salt stress
INTRODUCTION
Rice (Oryza sativa L.) is the most common staple food of 50% of the world’s population and provides 80% of the daily calorie needs in many populations, predominately in Asia (Hadiarto & Tran, 2010; Rasheed et al., 2020).
Crops are often exposed to unfavorable environmental conditions, such as abiotic stresses, which limits the yield. Salinity is one of the most important environmental factors limiting the productivity of crops. Most crops are sensitive to high concentrations of salts in the soil, and almost 30% of the world's potentially cultivable land is high salinity soil. This is a result of saline water irrigation and has been responsible for quantitative economic losses (Assouline et al., 2015; Qadir et al., 2014). High salinity concentration in the soil or the irrigation water can have a devastating effect on plant metabolism, disrupting cellular homeostasis and uncoupling major physiological and biochemical processes (Ahmad et al., 2010; Tanou et al., 2009). The negative impact of the stress associated with salinity on agriculture is expected to increase as a result of the projected global climate changes (Cardil et al., 2014; Lobell et al., 2011; Lobell et al., 2014; Papworth et al., 2015). Increases in salinity-related stress as a result of climate change are causing many problems in Asian countries such as China, Bangladesh, and South Korea (Miyan, 2015). During stress conditions caused by the drought and salinity, oxidative stress, directly or indirectly generated in plants, is one of the main drivers of damage to plant structure and functions. Oxidative stress results in damage of the cell membrane, alters membrane integrity and causes physiological and biochemical changes, which leads to acute metabolic disorders and eventually reduce crop productivity (Sharma & Zheng, 2019; Wang et al., 2019).
There are different approaches to mitigate salt-related stress on plants. Among them, traditional breeding and modern biotechnological tools are being used to prevent yield losses. Another approach includes the use of plant growth hormones, gibberellic acid (GA), cytokinin, and salicylic acid (SA), antioxidants (ascorbic acid), and osmoprotectants in foliar applications and seed treatments (Farooq et al., 2009; Senaratna et al., 2000). In addition, applications of biostimulants, such as seaweed extracts, are currently being studied to improve plant performance (Abdel Latef et al., 2017; Battacharyya et al., 2015; Craigie, 2011). Treatments based on natural sources, such as plant extracts, are eco-friendly and safe for sustainable agriculture and do not produce any adverse effects on the soil ecosystems.
The effect of biostimulants on the growth and quality of various plant species has been assessed (Chouliaras et al., 2009; Demir et al., 2006; Khan et al., 2009; Manna et al., 2012; Zheng et al., 2016). For example, various seaweed extracts have been reported to enhance plant tolerance against a wide range of abiotic stresses (Aziz et al., 2011; Santaniello et al., 2017; Shukla et al., 2017). There are also numerous reports on the benefits of applying seaweed extracts which have been shown to increase plant growth and yield parameters (Layek et al., 2018; Sharma et al., 2017). Until recently, only extracts of seaweed or related species have been used as treatments to potentially increase crop growth and reduce the damage associated with stress. There have only been a few studies on how extracts from natural plant sources such as leaves can be used to reduce stress damage.
The extracts of soybean (Glycine max (L.) Merr.) and Chinese chive (Allium tuberosum Rottler) leaves contain antioxidant compounds such as flavonoids, phenolic acids, and minerals and therefore may be effective in increasing crop yields by helping the crops cope better with environmental stress (Jang, 2017; Moon et al., 2003; Porter et al., 1985; Stutte & Park, 1973). In addition, Morsy et al. (2009) found that treatment with garlic or onion extracts significantly improved all the plant growth characteristics of the cucumber plant, i.e., the number of leaves, number of flowers, shoot and root length, and fresh and dry weight of the shoot and root system compared with untreated plants. However, few studies conducted to date have established whether other plant extracts or their chemical components could protect plants against abiotic stresses. Therefore, this study examined various plant extracts and their ability to reduce the stresses associated with salt in rice plants. Additionally, this study examined various physiological and biochemical parameters such as growth parameters, photosynthetic activity, and proline accumulation in rice plants after treatment with the selected plant extracts under salt stress conditions.
MATERIALS AND METHODS
Plant materials and extracts
For this study, tomato, onion, and Chinese chive leaf powder were purchased from Shinyoung Mall Co., Ltd. (South Korea), Chamduri Co. (South Korea), and Healthy Chotori Co., Ltd. (South Korea), respectively. Dried moringa (Moringa oleifera) leaves were purchased from Dooson Herb Co., Ltd. and then made into a powder using a coffee grinder (Proctor Silex E160B, Southern Pines, NC). Soybean leaves, soybean stems, and green tea (Camellia sinensis) leaves were collected from the Sunchon National University’s farm. Undaria pinnatifida, Saccharina japonica, Hizikia fusiforme, and Gracilaria verrucosa were collected from Goheung, Jeollanam-do, South Korea. These materials were dried in a drying oven at 45℃ for 3 days and made into a powder using a coffee grinder (Proctor Silex E160B, Southern Pines, NC).
To make the plant extracts, 50 g of the ground plant materials were mixed with 1000 mL of distilled water for 24 h. Thereafter, the extract was filtered through a Miracloth and completely evaporated using a vacuum dryer (Hanbaek Scientific Co. South Korea). Afterward, the extract was adjusted by distilled water to ensure that the final concentration was 50% (w/v), which was then further diluted with distilled water as needed to attain the proper concentrations for each experiment.
Salt stress treatments
Rice (cv. Hopumbyeo) plants were germinated in seed trays filled with a mixture of commercial paddy soil (SUNGHWA. Co., Ltd., Beolgyo, South Korea). the seed trays were placed in growth chambers (25℃/22℃ under a 14/10 h day/night regime, 150 μmol m–2s–1 photosynthetically active radiation and relative humidity of 60%). After 30 days of seed germination, roots of the seedlings were washed and seedlings were placed in conical tubes (15 mL). Each treatment was applied to conical tubes containing five plants each, and all experiments were replicated three times. Each tube was subjected to root treatments of either 100 mM NaCl or plant extracts at concentrations of 0.1%, 0.5%, 1%, or 3% combined with 100 mM NaCl. Control plants were subjected to the same salt stress but were not treated with any extracts. We chose a concentration of 100 mM NaCl after preliminary experiments showed that lower or higher concentrations caused either not enough or too much damage to plants. Seedlings were then placed in growth chambers (25℃/22℃ under a 14/10 h day/night regime, 150 μmol m–2s–1 photosynthetically active radiation and relative humidity of 60%) for six days. Plant extracts with a 1% concentration were selected for further study due to their significant reduction of salt stress on shoot fresh weight.
Rice growth and relative water contents after salt stress
Plant injury was investigated at 1, 2, 4, and 6 days after salt stress. Plant height and shoot fresh weight were measured after 6 days of salt stress. For relative water content, after harvesting, the samples were immediately weighed (Wf). The samples were then oven-dried at 105℃ for 24 hours and the dry weight was calculated (Wd). Then, their average was computed (Wt). Relative water content was calculated using the following formula:
Photosynthetic efficiency, chlorophyll and carotenoid contents after salt stress
Chlorophyll fluorescence analysis involves a non-invasive measurement of photosystem II (PSII). The quantum yield (Fv/Fm) and electron transport rate (ETR) of rice plants were measured after 4 and 6 days of salt stress. In vivo chlorophyll fluorescence of PSII was determined by using a portable pulse modulation fluorometer (PAM 2500, Walz, Effeltrich, Germany). Prior to measurements, the fronds were dark adapted for 15 min to expose all the antennae pigments.
Chlorophyll and carotenoid contents were assayed according to the procedure laid down by Hiscox & Israelstam (1979). The leaves (0.2 g) from each treatment were ground in N2 with a mortar and pestle, re-suspended with 5 mL of 100% methanol, and then centrifuged at 10,000 × g for 3 min. Spectrophotometric measurements of the absorbance of the resultant supernatant were made at 652.4, 665.2, and 470.0 nm. The chlorophyll and carotenoid contents were calculated using the following equations:
Determination of superoxide radical (O2−) and H2O2 contents after salt stress
The superoxide radical contents in the leaves were determined using the modified method of Elstner & Heupel (1976). A quantity of 200 mg of the leaves was homogenized with 1 mL of 50 mM phosphate buffer solution (pH 7.8) containing 2 mM hydroxylamine hydrochloride. After centrifugation at 12,000 × g for 30 min, 600 μl of the supernatants were mixed with 400 μl of phosphate buffer solution and incubated at 25℃ for 30 min. Then 1ml of 17 mM L-sulfanilic acid and 1 mL of 7 mM L-1-α- naphthylamine were added, and the mixture was shaken using an incubator (VS-1203PFC-L, LabTech. Namyang, South Korea) at room temperature for 30 min. The absorbance was determined colorimetrically at 530 nm.
The hydrogen peroxide (H2O2) level was measured colorimetrically as described by Jana & Choudhuri (1982). Hydrogen peroxide was extracted by homogenizing 0.2 g of leaves with 3 mL phosphate buffer (50 mM; pH, 6.8). The homogenate was centrifuged at 6000 × g, for 25 min. To determine the H2O2 levels, 3 mL of the extracted solution was mixed with 1 mL of 0.1% titanium chloride (Aldrich, St. Louis) in 20% (v/v) H2SO4, and the mixture was centrifuged, at 6000 × g for 15 min. The intensity of the yellow supernatant was measured at 410 nm. The H2O2 level was calculated using the extinction coefficient 0.28 mm–1cm–1.
Lipid peroxidation after salt stress
Lipid peroxidation was estimated by quantifying the amount of malondialdehyde (MDA) production using a slight modification of the thiobarbituric acid (TBA) method, as previously described by Buege & Aust (1978). Each leaf (0.5 g) was ground using a mortar and pestle with 5 mL 0.5% TBA in 20% trichloroacetic acid. The homogenate was then centrifuged at 20,000 × g for 15 min, and the supernatant was collected. The supernatant was heated in a boiling water bath for 25 min and allowed to cool in an ice bath. After additional centrifugation at 20,000 × g for 15 min, the resulting supernatant was used for the spectrophotometric determination of MDA. The absorbance at 532 nm for each sample was recorded and subtracted for nonspecific turbidity at 600 nm. MDA concentrations were calculated using a molar extinction coefficient of 156 mm–1cm–1 and the following formula: MDA (micromoles per gram dry weight) = [(A532 – A600)/156] × 103 × dilution factor (Du & Bramlage, 1992).
Proline and sugar accumulation after salt stress
Proline was extracted by grinding 1 g of frozen plant material using a mortar and pestle. The grinding material was homogenized with 5 mL of 3% sulfosalicylic acid and the debris was removed by centrifugation at 2000 × g for 10 minutes. 3 mL of the extract was reacted with 3 mL of glacial acetic acid and 3 mL color-developing solution (125 mg ninhydrin warmed to be dissolved in 3 mL glacial acetic acid and 2 mL 6 M phosphoric acid) for 30 minutes at 100℃, and the reaction was terminated in an ice bath. The reaction mixtures were mixed with 5 mL of toluene. The chromophore containing toluene was aspirated from the aqueous phase and warmed up to room temperature for 24 hours. The amount of proline was determined with a spectrophotometer at 520 nm.
The soluble sugar of the leaves was extracted and quantified by a modified method of Xu et al. (2015). 50 mg of ground sample was extracted with 1 mL of 80% (v/v) ethanol at 80℃ for 30 min, followed by centrifugation at 14,000 × g for 10 min. The residue was extracted two more times using 80% ethanol. The three supernatants were combined and 80% ethanol was added to bring the total volume to 5 mL. The soluble sugar content was determined spectrophotometrically at A620 nm wavelength. The sucrose content was determined spectrophotometrically at A480 nm wavelength.
Statistical analysis
The experiments were conducted in a completely randomized design with three replications. Some data were expressed as percentages of the untreated control for easy comparisons between the treatments. Data were analyzed using the analysis of variance (ANOVA) procedure using the Statistical Analysis Systems software 7 (SAS 2000). There were significant treatments x concentrations by factorial interaction. The means were separated using Duncan’s Multiple Range Test (p = 0.05).
RESULTAS AND DISCUSSION
Effects of plant extracts on rice injury and growth under salt stress
To select the plant extracts that re effective in reducing the damage associated with salt stress, we treated rice plants with various plant extracts (Table 1). Injuries to rice plants identified based on visual symptoms increased over time under salt stress regardless of the type of plant extract used. Chinese chive, onion, and tomato extracts at certain concentrations reduced the injuries caused by salt stress; however, other concentrations were not effective. However, most extracts, such as soybean leaf, soybean stem, moringa, and Undaria pinnatifida, effectively reduced injuries caused due to salt stress. Plant height was not significantly reduced by salt stress in both the control plants and the treated plants. The shoot fresh weight of rice plants was higher when treated with Chinese chive, tomato, and moringa extracts at some concentrations when compared with the shoot fresh weight of the control plants under salt stress. The shoot fresh weight of the rice plants after the onion and green tea extract treatments was similar to the shoot fresh weight of the control plants. However, the shoot fresh weight of plants treated with other extracts such as soybean leaf, soybean stem, moringa, and Undaria pinnatifida was higher than the shoot fresh weight of the control plants under salt stress. Hence, we selected the soybean leaf, soybean stem, moringa, and Undaria pinnatifida extracts for further studies.
Table 1.
Effects of various plant extracts on rice injury, plant height, and shoot fresh weight under salt stress.
Extract |
Con.* (%) | Leaf injury (%)* |
Plant height (cm) |
Shoot F.W* (g/plant) | |||
1 DAT | 2 DAT | 4 DAT | 6 DAT | ||||
Non-stress | 0d | 0g | 0e | 0k | 24.3 (100)a-f | 0.230 (100)a | |
Salt stress | 100 mM | 10c | 30a-c | 40a-c | 65a-d | 23.6 (97.1)b-f | 0.130 (56.5)k-o |
Soybean leaf | 0.1 | 0d | 10e-g | 20c-e | 20i-k | 24.8 (102.1)a-f | 0.195 (84.8)b |
0.5 | 0d | 10e-g | 20c-e | 20i-k | 25.7 (105.8)a-e | 0.224 (97.4)a | |
1 | 10c | 10e-g | 20c-e | 30g-j | 26.6 (109.5)ab | 0.178 (77.4)b-d | |
3 | 10c | 25b-d | 45ab | 60a-e | 24.6 (101.2)a-f | 0.139 (60.4)h-n | |
Soybean stem | 0.1 | 10c | 10e-g | 20c-e | 30g-j | 25.9 (106.6)a-e | 0.152 (66.1)e-k |
0.5 | 10c | 10e-g | 20c-e | 25h-j | 25.3 (104.1)a-f | 0.188 (81.7)b | |
1 | 10c | 10e-g | 20c-e | 25h-j | 25.9 (106.6)a-e | 0.217 (94.3)a | |
3 | 0d | 10e-g | 15de | 20i-k | 26.9 (110.7)a | 0.221 (96.1)a | |
Chinese chive | 0.1 | 0d | 10e-g | 20c-e | 50c-g | 23.4 (96.6)c-f | 0.154 (67.0)e-j |
0.5 | 10c | 20c-e | 40a-c | 50c-g | 25.0 (102.9)a-f | 0.174 (75.6)b-e | |
1 | 10c | 30a-c | 45a-e | 70a-c | 25.1 (103.3)a-f | 0.149 (64.8)f-l | |
3 | 30a | 40a | 50a | 80a | 26.1 (107.4)a-c | 0.133 (57.8)j-o | |
Onion | 0.1 | 0d | 0g | 30a-d | 45d-h | 22.9 (94.2)d-f | 0.125 (53.4)m-p |
0.5 | 0d | 0g | 30a-d | 50c-g | 23.4 (96.3)c-f | 0.130 (56.5)k-o | |
1 | 10c | 20c-e | 40a-c | 60a-e | 23.7 (97.5)b-f | 0.135 (58.7)i-o | |
3 | 10c | 20c-e | 50a | 80a | 26.3 (108.2)a-c | 0.134 (58.2)i-o | |
Tomato | 0.1 | 0d | 10e-g | 20c-e | 50c-g | 24.1 (99.2)a-f | 0.134 (58.3)i-o |
0.5 | 0d | 10e-g | 30a-d | 60a-e | 25.5 (104.9)a-f | 0.166 (71.2)c-f | |
1 | 10c | 25b-d | 40a-c | 60a-e | 23.8 (97.9)a-f | 0.146 (63.5)f-m | |
3 | 25ab | 35ab | 45ab | 75ab | 24.0 (98.8)a-f | 0.144 (62.6)f-m | |
Camellia sinensis | 0.1 | 0d | 10e-g | 20c-e | 40e-i | 22.8 (93.8)ef | 0.107 (46.5)p |
0.5 | 0d | 10e-g | 20c-e | 47c-h | 22.5 (92.5)f | 0.142 (61.7)g-n | |
1 | 10c | 10e-g | 20c-e | 47c-h | 25.1 (103.3)a-f | 0.129 (56.1)l-o | |
3 | 5cd | 10e-g | 30a-d | 55b-f | 23.5 (96.7)b-f | 0.116 (50.4)op | |
Moringa oleifera | 0.1 | 0d | 5fg | 10de | 30g-j | 23.5 (96.7)b-f | 0.149 (64.8)f-l |
0.5 | 0d | 10e-g | 20c-e | 30g-j | 26.1 (107.4)a-d | 0.178 (77.4)b-d | |
1 | 0d | 15d-f | 25b-d | 40e-i | 24.0 (98.8)a-f | 0.121 (52.6)n-p | |
3 | 20b | 30a-c | 50a | 80a | 25.3 (104.1)a-f | 0.108 (47.0)p | |
Undaria pinnatifida | 0.1 | 5cd | 10e-g | 20c-e | 30g-j | 26.0 (107.0)a-d | 0.161 (70.0)c-h |
0.5 | 5cd | 10e-g | 20c-e | 30g-j | 25.6 (105.3)a-f | 0.155 (67.4)e-j | |
1 | 5cd | 10e-g | 15de | 20i-k | 25.8 (106.2)a-e | 0.182 (79.1)bc | |
3 | 5cd | 10e-g | 15de | 20i-k | 24.0 (98.8)a-f | 0.175 (76.1)b-e | |
Saccharina japonica | 0.1 | 0d | 10e-g | 30a-d | 50c-g | 25.4 (104.5)a-f | 0.150 (65.2)f-l |
0.5 | 0d | 10e-g | 30a-d | 50c-g | 24.6 (101.2)a-f | 0.161 (70.0)c-h | |
1 | 10c | 20c-e | 40a-c | 45d-h | 25.3 (104.1)a-f | 0.162 (70.4)c-g | |
3 | 10c | 15d-f | 25b-d | 40e-i | 25.3 (104.1)a-f | 0.163 (70.9)c-g | |
Hizikia fusiforme | 0.1 | 0d | 10e-g | 20c-e | 40e-i | 23.9 (96.7)a-f | 0.139 (60.4)h-n |
0.5 | 5cd | 10e-g | 30a-d | 47c-h | 24.4 (100.4)a-f | 0.153 (66.5)e-j | |
1 | 5cd | 10e-g | 30a-d | 50c-g | 24.7 (101.6)a-f | 0.173 (75.2)b-e | |
3 | 0d | 10e-g | 30a-d | 45d-h | 24.7 (101.6)a-f | 0.146 (63.5)f-m | |
Gracilaria verrucosa | 0.1 | 0d | 10e-g | 15de | 25h-j | 24.4 (100.4)a-f | 0.143 (62.2)f-n |
0.5 | 0d | 5fg | 20c-e | 30g-j | 24.2 (99.6)a-f | 0.164 (71.3)c-g | |
1 | 0d | 5fg | 10de | 15jk | 25.0 (102.9)a-f | 0.174 (75.7)b-e | |
3 | 10c | 20c-e | 20c-e | 35f-j | 25.8 (106.2)a-e | 0.156 (67.8)d-i | |
Treatment | ** | *** | |||||
Concentration | 0.13 | *** | |||||
Treatment × Concentration | 0.44 | *** |
Out of the eleven extracts used, onion and green tea extract treatment of crops under salt stress did not reduce rice damage. All other extracts, including Undaria pinnatifida, Saccharina japonica, Hizikia fusiforme, and Gracilaria verrucose extracts which originated from sea plants, were effective in reducing salt stress. To date, extracts made from seaweed species have been studied extensively and have been found to be effective in alleviating a variety of abiotic stresses, including drought, salinity, and temperature (Craigie, 2011). Seaweed extract also increases the endogenous concentrations of stress-related molecules, such as cytokinins, proline, and antioxidants in treated plants (Fan et al., 2013).
Another effective extract used in this study came from moringa, which ameliorated salt stress. However, the effectiveness was lower when compared to other plant extracts. In another similar study, foliar-applied moringa leaf extract could ameliorate salinity-induced adverse effects by activating the antioxidant defense system and decreasing the accumulation of Na+ and Cl- in the shoots under moderate saline conditions (Yasmeen et al., 2013). Chaetomorpha antennina aqueous extracts were also found to be capable of alleviating salt stress in rice plants in an efficient, eco-friendly, and economical manner (Chanthini et al., 2019). Acacia dealbata bark extract significantly increased the height as well as the leaves, roots, and total biomass of plants in soils irrigated with NaCl solution (120 mmol L−1) (Lorenzo et al., 2019). Although many plant extracts have been studied for their ability to reduce stress damage, the extracts made from soybean leaf, soybean stems and Chinese chive used in this study, have not been studied in detail earlier.
Effects of selected plant extracts on relative water content under salt stress
Relative water content is an integrated measurement of plant water status. It is an indicator of the physiological consequences of cellular water deficit and represents the variations in the water potential and turgor potential of the plants (Gupta et al., 2020). Water stress is one of several factors that negatively affect the relative water content, turgor pressure, and transpiration in many crops (Rao & Chaitanya, 2016). Thus, to determine the water content of the leaves under salt stress, rice plants were treated with the selected plant extracts (Fig. 1). After 4 days of salt stress, the water content in the leaves did not vary regardless of the type of plant extract treatments used. However, after 6 days of salt stress, the water content in the leaves of the treated plants was significantly higher than that in the control plants. All the selected extracts except Gracilaria verrucose helped plants retain the water content in their leaves. Under salt stress, the application of certain plant extracts may increase the water content in the leaves of rice plants, and this effect becomes more noticeable after six days. These results suggest that the selected plant extracts have a consistent and positive impact on water content in rice plants under extended salt stress conditions.
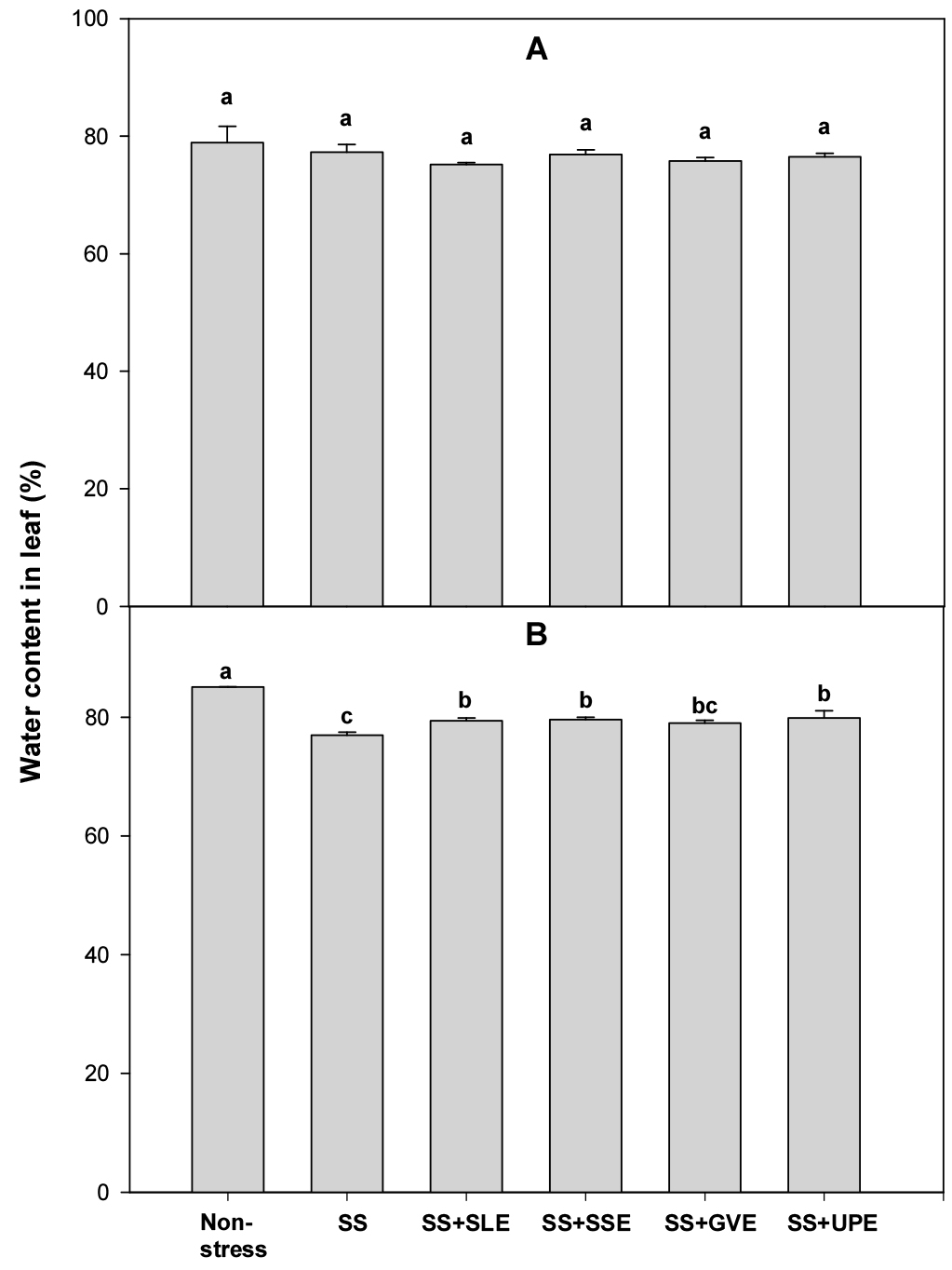
Fig. 1.
Effects of selected extracts on leaf water content at 4 (A) and 6 (B) days after treatment with extracts at 1% concentration of salt-stressed plants (SS, Salt stress; SS+SLE, Salt stress+Soybean leaf extract; SS+SSE, Salt stress+Soybean stem extract; SS+GVE, Salt stress+G. verrucosa extract; SS+UPE, Salt stress+ U. pinnatifida extract). Means with different letters are significantly different according to Duncan’s Multiple Range Test at 5% level. Error bars of the mean (±SE) result from three replicates.
Effects of selected plant extracts on photosynthetic efficiency and pigments under salt stress
One of the major consequences of water deficit in higher plants is the decrease or suppression of photosynthesis (Nezhadahmadi et al., 2013). Fv/Fm and ETR can be used as indicators to evaluate the photosynthetic activity of plant leaves (Haimeirong et al., 2002). The photosynthetic efficiency (Fv/Fm and ETR) of the rice plants under salt stress was measured after treatment with the selected plant extracts (Fig. 2). Fv/Fm was significantly higher in all the treated plants compared with the control plants after 4 and 6 days of salt stress. ETR was also significantly higher in all the treated plants than in the control plants after 6 days of salt stress, but showed no difference after 4 days. The photosynthetic capacities of leaves are important factors that reduce yield in susceptible rice genotypes under drought stress conditions (Zhu et al., 2020). In addition, drought or salt stress lowers the rate of photosynthesis, and alters the distribution and metabolism of carbon in plants, leading to depleted energy and decreased yield (Cuellar-Ortiz et al., 2008). This study, too, suggests that the reduction of the water content and reduced photosynthetic efficiency resulting in lower shoot fresh weight may be caused by salt stress.
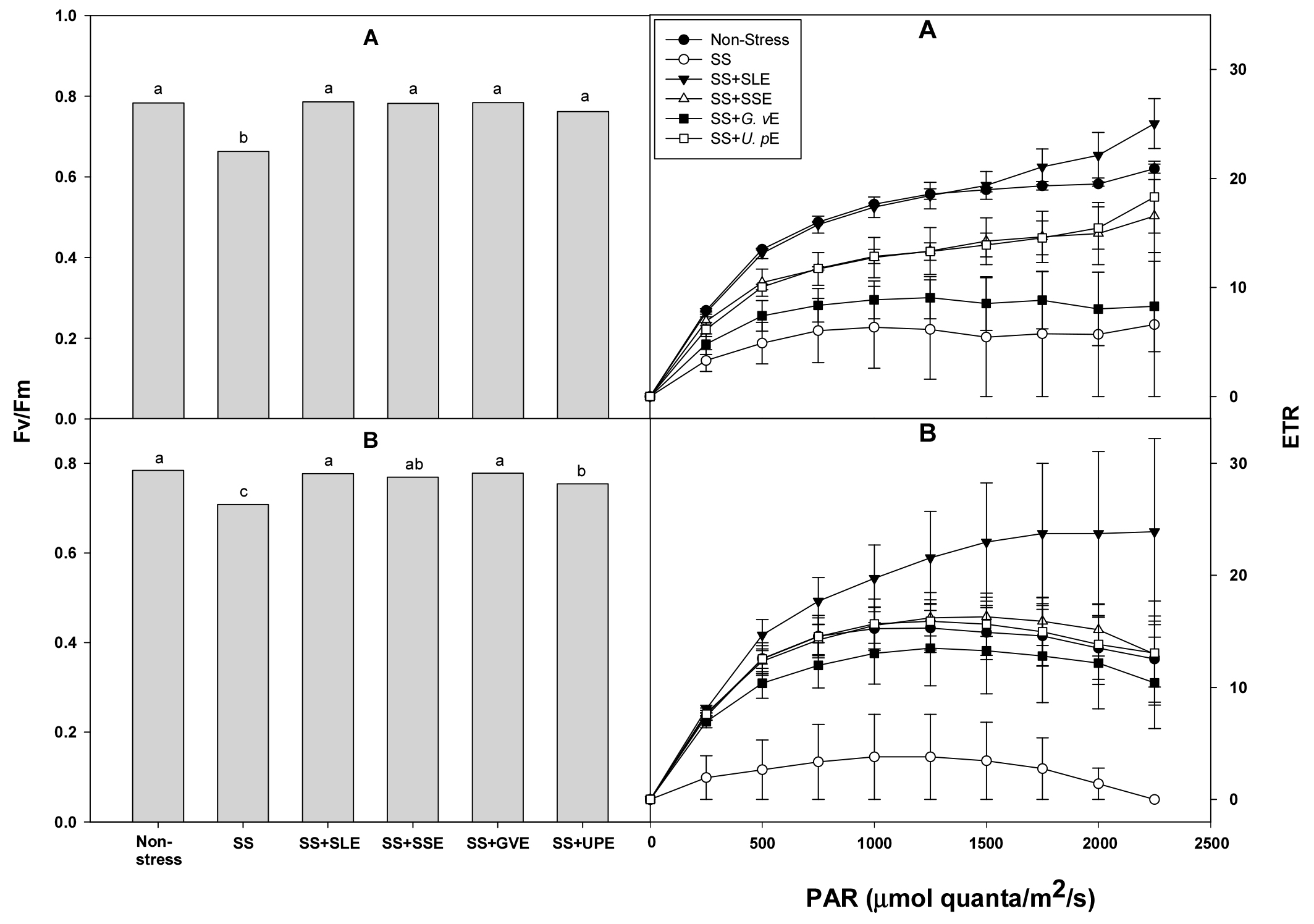
Fig. 2.
Effects of selected plant extracts at 1% concentration on chlorophyll a fluorescence (Fv/Fm) and ETR at 4 (A) and 6 (B) days after treatment under salt stress (SS, Salt stress; SS+SLE, Salt stress+Soybean leaf extract; SS+SSE, Salt stress+Soybean stem extract; SS+GVE, Salt stress+G. verrucosa extract; SS+UPE, Salt stress+U. pinnatifida extract). Means with different letters are significantly different according to Duncan’s Multiple Range Test at 5% level. Error bars of the mean (±SE) result from three replicates.
Chlorophyll, the primary pigment in photosynthesis and is found in the chloroplasts (Rahdari et al., 2012). The decrease in chlorophyll content under stress conditions has been considered a typical symptom of pigment photooxidation and chlorophyll degradation (Anjum et al., 2011). In addition, carotenoids are the essential components for photoprotection and act as precursors in directing the signals for the growth of plants under stress conditions.
The chlorophyll and carotenoid contents of the plants under salt stress were measured after treatment with the selected plant extracts (Fig. 3). The chlorophyll content after 4 days of salt stress was higher (57-85%) in all the treated plants when compared with the control plants. When compared with the control plants, the chlorophyll content after 6 days of salt stress was also higher in all the treated plants except for those treated with soybean leaf extract. The carotenoid content after 4 days of salt stress was significantly higher (8-16%) in all the treated plants except for those treated with Gracilaria verrucose extract. However, there were no significant differences between all the treated plants and the control plants after 6 days of salt stress. Overall, the chlorophyll and carotenoid contents varied based on the types and levels of stress that the plants were subjected to. Another similar study showed that the degree to which chlorophyll content decreases varies with the duration and severity of stress (Zhang & Kirkham, 1996). In plant extract study, seaweed extract treatments produced an increase in chlorophyll content in the treated plants. This effect has been observed in a wide range of crops, including grapevine and strawberry (Blunden et al., 1997; Fan et al., 2013; Jannin et al., 2013; Mancuso et al., 2006; Sivasankari et al., 2006; Spinelli et al., 2010).
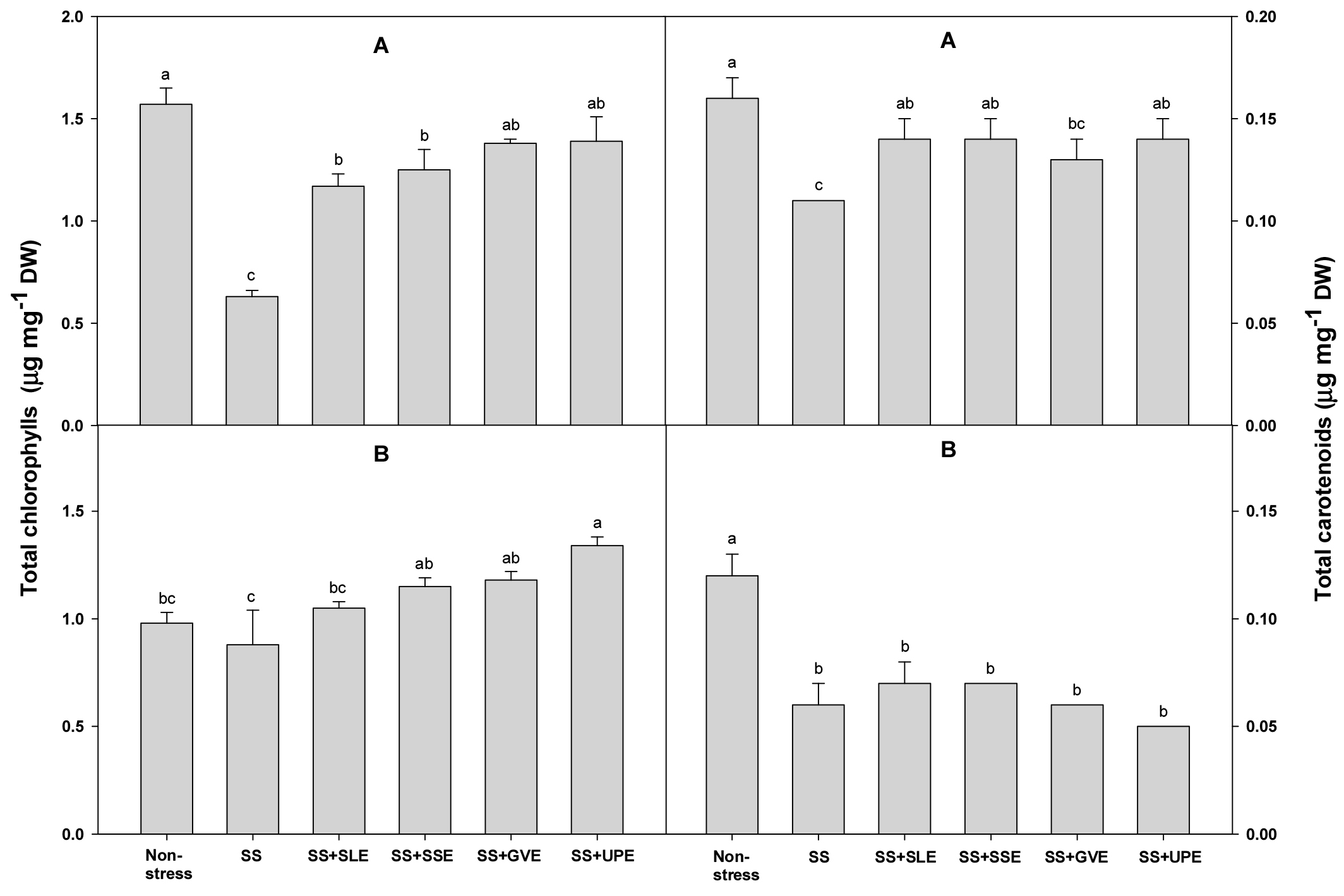
Fig. 3.
Effects of selected plant extracts at 1% concentration on total chlorophyll and carotenoid contents at 4 (A) and 6 (B) days after treatment under salt stress (SS, Salt stress; SS+SLE, Salt stress+Soybean leaf extract; SS+SSE, Salt stress+Soybean stem extract; SS+GVE, Salt stress+G. verrucosa extract; SS+UPE, Salt stress+U. pinnatifida extract). Means with different letters are significantly different according to Duncan’s Multiple Range Test at 5% level. Error bars of the mean (±SE) result from three replicates.
Effects of selected plant extracts on reactive oxygen species and lipid peroxidation under salt stress
Excess amounts of reactive oxygen species (ROS) such as O2−, and H2O2 are produced during times of salt stress due to the adverse effects of the photo-respiratory pathways pathways (Gill & Tuteja, 2010). ROS are highly toxic radicals that damage various cellular components such as proteins and membranes and disrupt cellular processes like lipid peroxidation, ultimately leading to cellular death (Gill & Tuteja, 2010). Hence, we measured the O2− and H2O2 levels to confirm the effectiveness of treatment with plant extracts given to rice plants that were subjected to salt stress (Fig. 4). The levels of O2− of all the treated plants and control plants after 4 days of salt stress were similar. At 6 days of salt stress, the levels of O2−were lower only in the plants treated with the Undaria pinnatifida extract. Plants treated with the extracts of soybean stem and Gracilaria verrucose had a lower H2O2 level after both 4 and 6 days of salt stress when compared with the control plants. In this study, it is suggested that the application of certain plant extracts, especially from soybean stem, onion, and Gracilaria verrucose, can reduce the levels of reactive oxygen species, showcasing their potential as biostimulants or stress-alleviating agents in the context of salt stress.
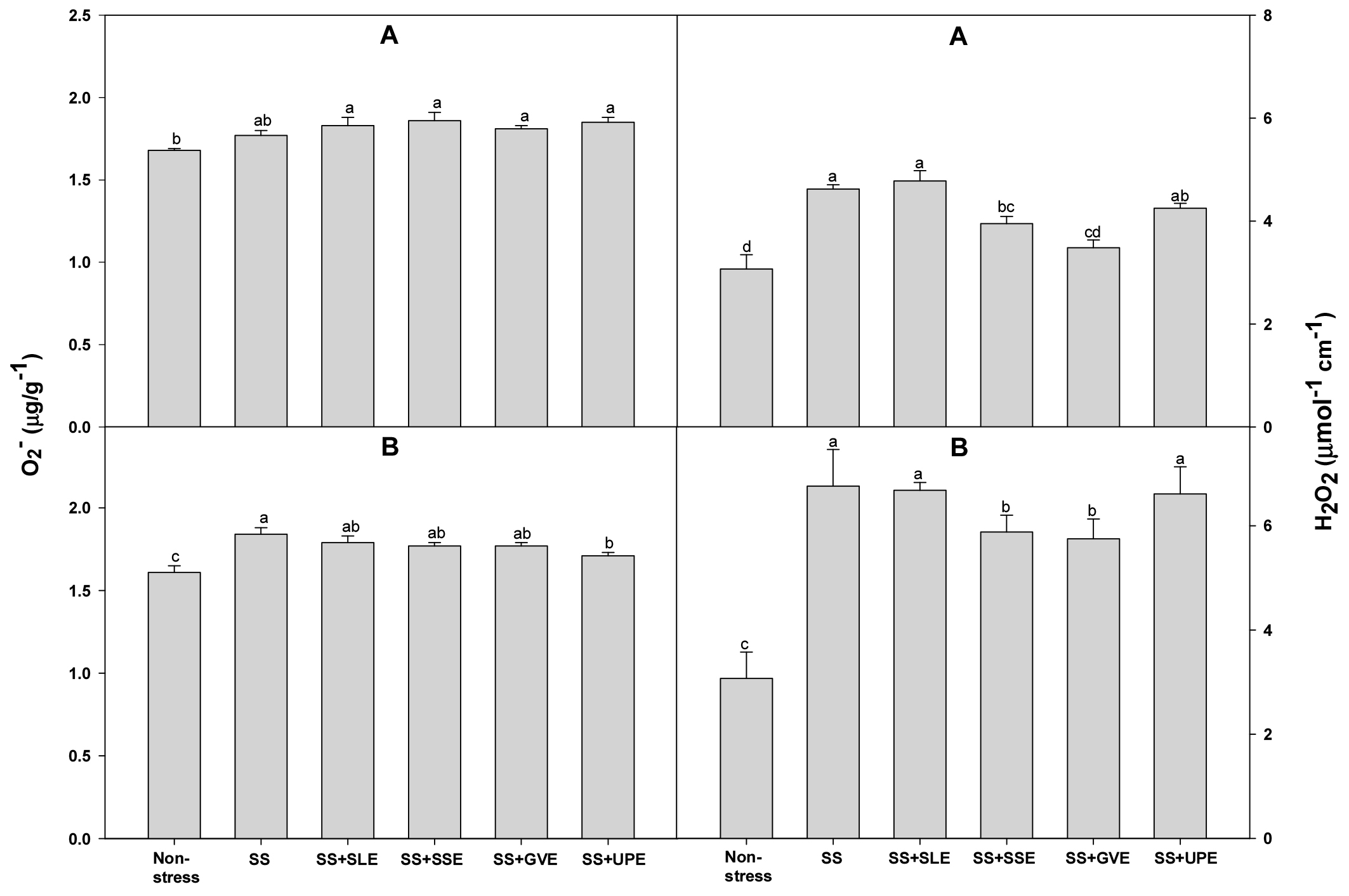
Fig. 4.
Effects of selected plant extracts at 1% concentration on O2- and H2O2 contents at 4 (A) and 6 (B) days after treatment under salt stress (SS, Salt stress; SS+SLE, Salt stress+Soybean leaf extract; SS+SSE, Salt stress+Soybean stem extract; SS+GVE, Salt stress+G. verrucosa extract; SS+UPE, Salt stress+U. pinnatifida extract). Means with different letters are significantly different according to Duncan’s Multiple Range Test at 5% level. Error bars of the mean (±SE) result from three replicates.
Lipid peroxidation is measured as the amount of MDA produced when polyunsaturated fatty acids in the membrane undergo oxidative degradation due to the accumulation of ROS (Hernandez et al., 2000). We determined the amount of MDA after salt stress (Fig. 5). The levels of MDA after 4 days of salt stress were lower (14-22%) in the plants treated with soybean leaf and soybean stem extracts than in the control plants. However, the levels of MDA after 6 days of salt stress were lower (45-51%) in all the treated plants when compared with the control plants.
In an earlier study, the MDA levels in rice seedlings increased significantly with increasing stress levels (Amirjani, 2010). Rice and rapeseed seedlings under stress conditions also showed significant increases in H2O2 and MDA content (Amirjani, 2010; Hasanuzzaman & Fujita, 2011). However, Lessonia nigrescens treatments decreased lipid peroxidation and alleviated the salt-induced loss of chlorophyll content (Zou et al., 2019). This study suggests that the application of certain plant extracts, especially from soybean leaf, soybean stem, and onion, can mitigate lipid peroxidation, showcasing their potential as protective agents against oxidative damage in the context of salt stress.
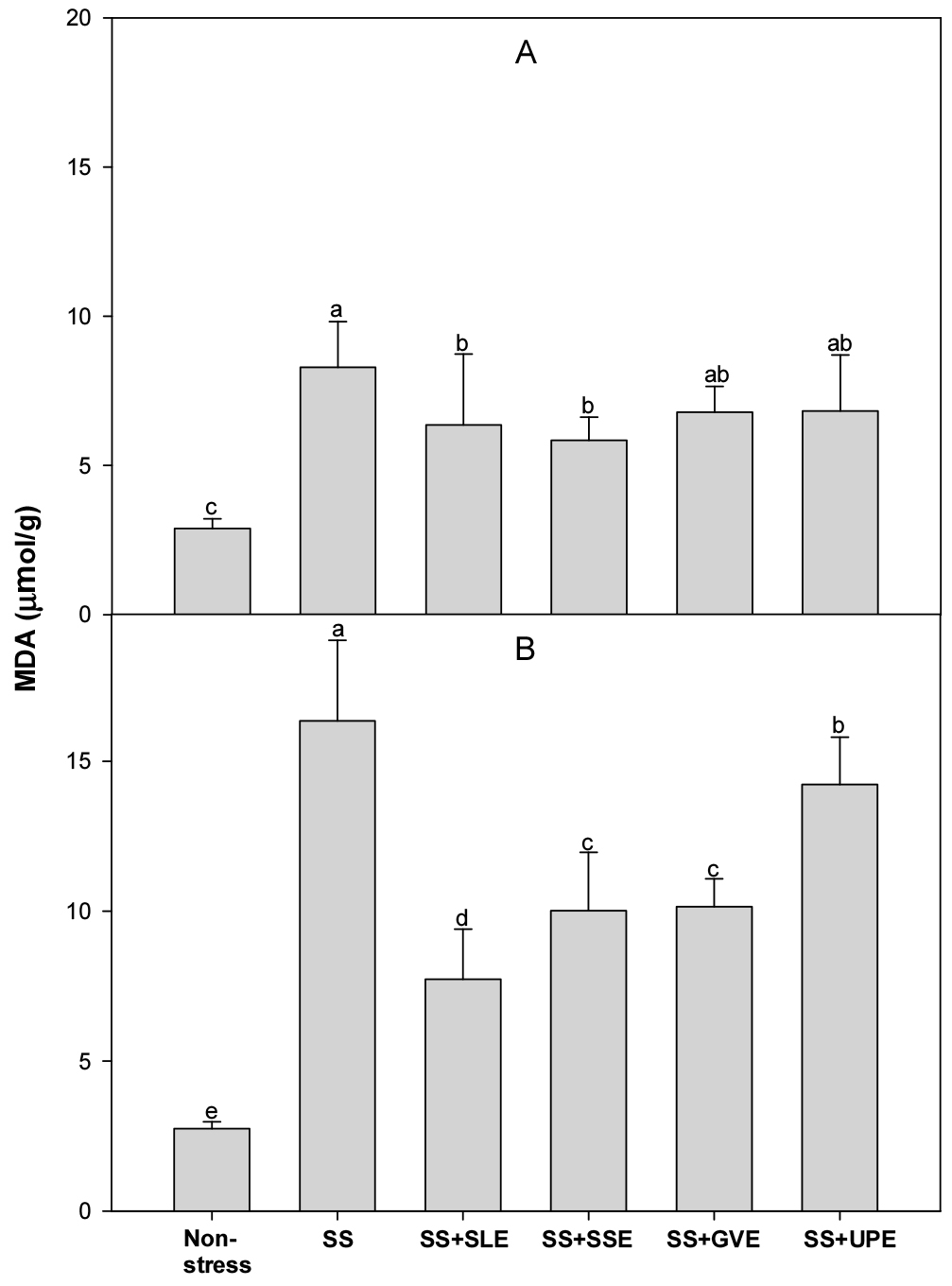
Fig. 5.
Effects of selected plant extracts on MDA production at 4 (A) and 6 (B) days after treatment with extracts at 1% concentration of salt-stressed plants (SS, Salt stress; SS+SLE, Salt stress+Soybean leaf extract; SS+SSE, Salt stress+Soybean stem extract; SS+GVE, Salt stress+G. verrucosa extract; SS+UPE, Salt stress+U. pinnatifida extract). Means with different letters are significantly different according to Duncan’s Multiple Range Test at 5% level. Error bars of the mean (±SE) result from three replicates.
Effects of selected plant extracts on proline and sugar accumulation under salt stress
Proline accumulation is a well-known metabolic response of plants to salt and other stresses (Szabados & Savouré, 2010). Hence, to confirm the effectiveness of the plant extract treatments on rice plants that experienced salt stress, we measured the levels of proline (Fig. 6). There was no significant difference in the proline levels after 4 days of salt stress between the treated plants and the control plants. However, after 6 days of salt stress, the proline levels were significantly higher in all the treated plants compared to those of the control plants. Based on the results of this study, we believe that the accumulation of proline is a key indicator of the response of rice plants to salt stress. Some studies have shown that proline accumulation is generally an indicator of stress and is associated with stress susceptibility (Hanson et al., 1977). Additionally, earlier studies have shown that the proline concentration in rice increased significantly during drought and salt stress (Mostajeran & Rahimi-Eichi, 2009; Nounjan & Theerakulpisut, 2012). However, Bing-Sheng et al. (2014) have suggested that proline accumulation is not correlated with salt, alkaline, and osmotic stresses in rice.
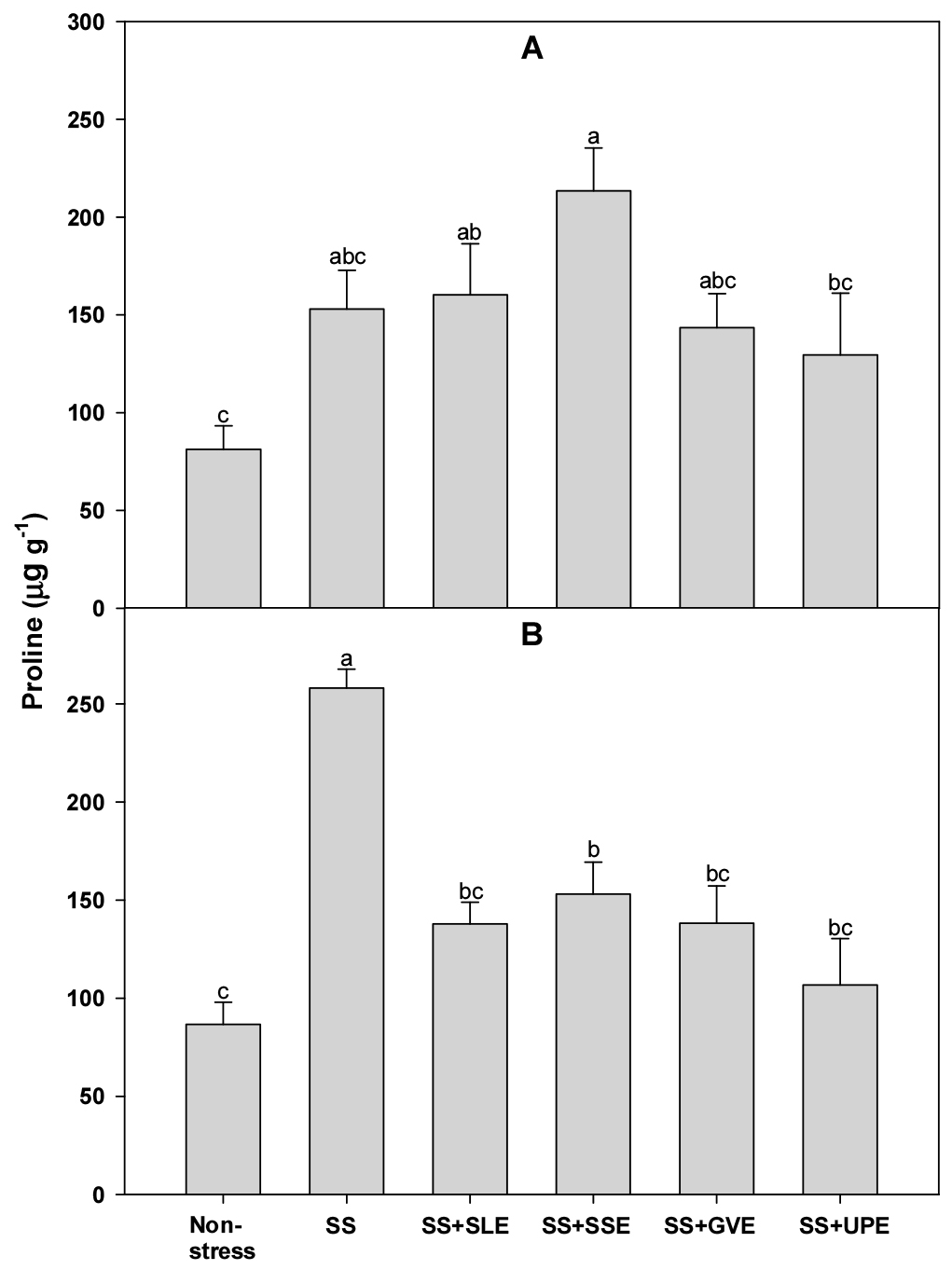
Fig. 6.
Effects of selected plant extracts on proline contents at 4 (A) and 6 (B) days after treatment with extracts at 1% concentration of salt-stressed plants (SS, Salt stress; SS+SLE, Salt stress+Soybean leaf extract; SS+SSE, Salt stress+Soybean stem extract; SS+GVE, Salt stress+G. verrucosa extract; SS+UPE, Salt stress+U. pinnatifida extract). Means with different letters are significantly different according to Duncan’s Multiple Range Test at 5% level. Error bars of the mean (±SE) result from three replicates.
Soluble sugars (sucrose, glucose, and fructose) play an important role in maintaining the overall structure and growth of plants (Rosa et al., 2009). Hence, we measured the levels of soluble sugars in plants treated with the extracts and the control plants under salt stress (Fig. 7). The soluble sugar content after 4 days of salt stress was significantly lower (5-16%) in plants treated with soybean stem and the Gracilaria verrucosa extracts than in the control plants. However, after 6 days of salt stress, the soluble sugar content was significantly lower (9-26%) in all the treated plants when compared with that in the control plants. This suggests that the selected plant extracts may impact the plant's ability to maintain soluble sugar levels under prolonged salt stress. Interestingly, in salt stress conditions, the plants treated with extracts exhibited lower proline and soluble sugar accumulation compared to control plants. This suggests that the application of plant extracts might modulate these metabolic responses, potentially contributing to improved stress tolerance. The observed reduction in proline and soluble sugar accumulation in treated plants may signify a more efficient stress response mechanism that minimizes the negative impact of salt stress.
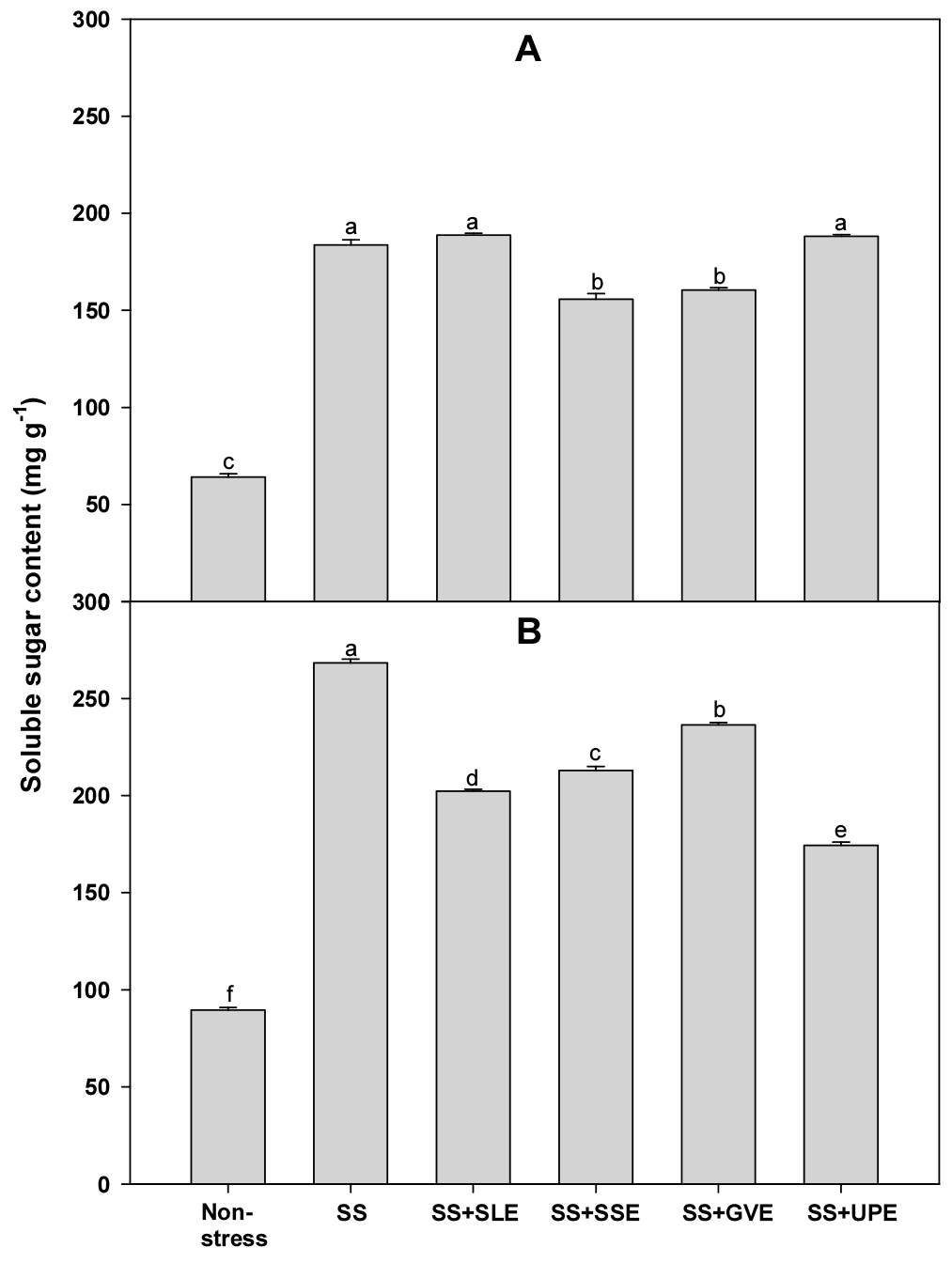
Fig. 7.
Effects of selected plant extracts on soluble sugar contents at 4 (A) and 6 (B) days after treatment with extracts at 1% concentration of salt-stressed plants (SS, Salt stress; SS+SLE, Salt stress+Soybean leaf extract; SS+SSE, Salt stress+Soybean stem extract; SS+GVE, Salt stress+G. verrucosa extract; SS+UPE, Salt stress+U. pinnatifida extract). Means with different letters are significantly different according to Duncan’s Multiple Range Test at 5% level. Error bars of the mean (±SE) result from three replicates.
In conclusions, out of the eleven extracts used, we selected four plant extracts (soybean leaf, soybean stem, moringa, and Undaria pinnatifida extracts reduced salt stress by 20-40% compared to the control. Although the effectiveness of the extracts varied depending on the levels of stress and the extract concentrations, the overall levels of effectiveness were similar across all the plant extracts used. Generally, most parameters such as water content, photosynthetic efficiency (Fv/Fm and ETR), and pigments (chlorophyll and carotenoid) were higher in the plants treated with extracts when compared with the control plants. However, the levels of reactive oxygen species, MDA, proline, and soluble sugars were lower in the plants treated with extracts than in the control plants. Thus, the selected plant extracts can be used to alleviate the adverse effects of salt stress.